The ozone hole
Ozone-depleting substances are present throughout the stratospheric ozone layer because they are transported over great distances by atmospheric air motions. The severe depletion of the Antarctic ozone layer known as the “ozone hole” occurs because of the special meteorological and chemical conditions that exist there and nowhere else on the globe. The very low winter temperatures in the Antarctic stratosphere cause polar stratospheric clouds (PSCs) to form. Special reactions that occur on PSCs, combined with the isolation of polar stratospheric air in the polar vortex, allow chlorine and bromine reactions to produce the ozone hole in Antarctic springtime.
History
With the increasing emissions of chlorofluorocarbon (CFCs) gases, used in many household products such as refrigerators, cleaning solvents, air conditioners, and spray cans, the scientific world became alerted in the mid 1970s about the possible threat to the ozone layer from those gases. In 1974, Mario Molina and Sherwood Rowland published in Nature such a warning, which combined two important findings from other researchers: (i) James Lovelock and colleagues could demonstrate in 1973 that CFC gases had already spread out globally in the atmosphere with a self-developed higly sensitive device for measuring extremely low organic gas contents in the atmosphere, and (ii) Richard Stolarski and Ralph Cicerone showed that free chlorine atoms in the atmosphere can decompose ozone in similar ways (catalytically) as nitrogen oxides do (this latter had already been proposed by Paul Crutzen in 1971).
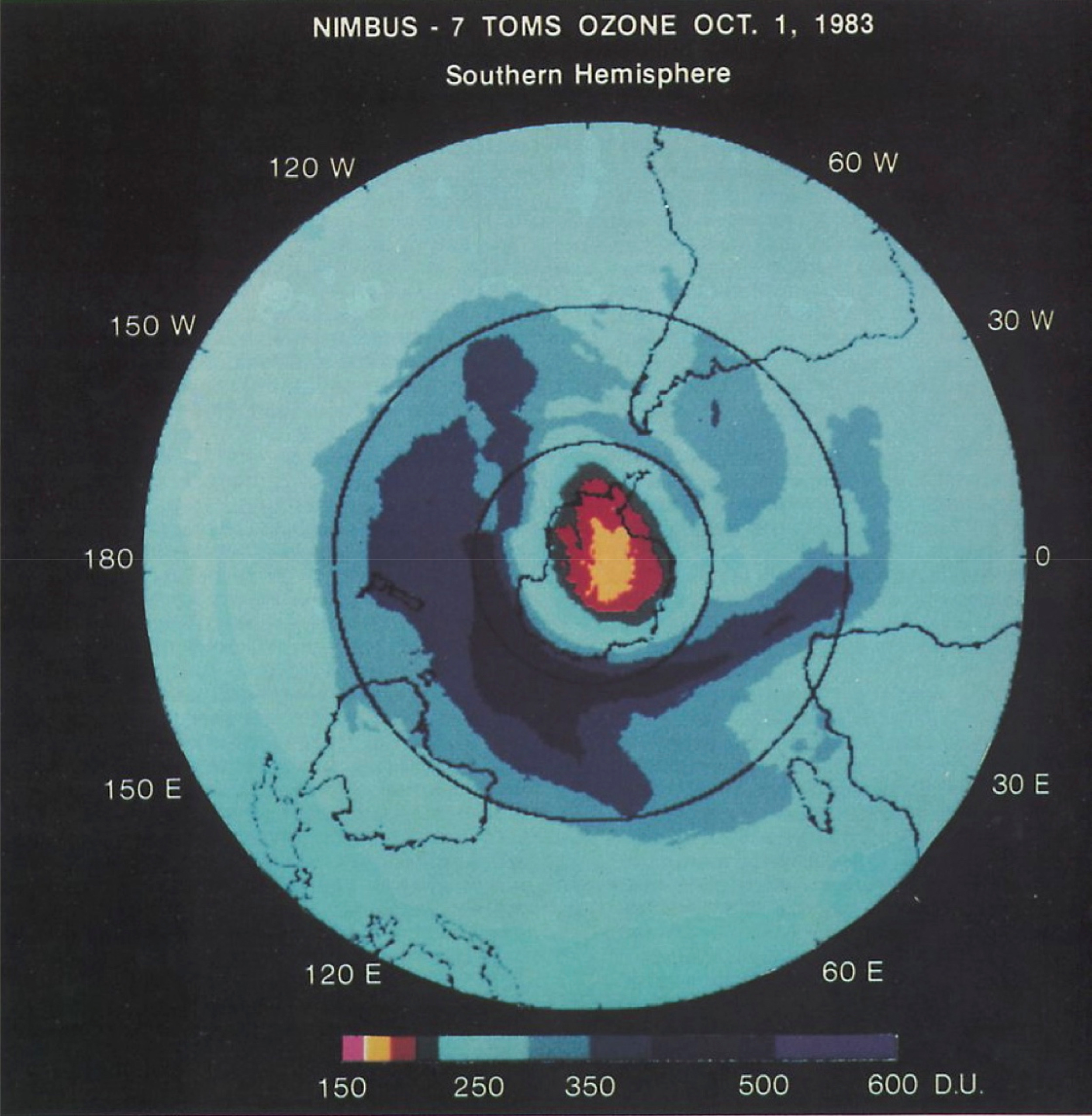
However, the drastic depletion of the October total ozone amounts over the British Antarctic station Halley Bay, as reported by Joseph Farman and colleagues in 1985, was undoubtedly a total surprise. In August 1985, at a conference in Prague, Bhartia et al. (1985) showed the first map of the Antarctic Ozone Hole produced by the Total Ozone Mapping Spectrometer (TOMS) satellite instrument (see Fig. 1). These results confirmed the findings of Farman and his colleagues and showed that the decrease they had observed was a continent scale phenomenon.
Finally, the images of the ozone hole produced from satellites captured the attention of public and media all over the world, and led to scientific field campaigns using aircraft, balloon, and advanced ground-based instruments deployed over Antarctica, coupled with new laboratory measurements, to better understand the chemistry of the stratospheric ozone layer. Though, plans to phase out the Ozone Depleting Substances (ODS) had started before the discovery of the ozone hole, and arguably the signing of the Montreal Protocol in 1987 that actually phased out and banned the CFCs and other ODS, was not directly influenced by it, there is no doubt that the discovery of the ozone hole greatly influenced the public and policy makers in accepting actions recommended by the signatories of the Montreal Protocol, despite its impact on a multi-billion-dollar global chemical industry.
A nice overview paper on the discovery of the Antarctic ozone hole can be found here.
Processes
The surprisingly rapid depletion of the ozone layer over Antarctica could not be explained by transport processes or by gas phase chemical reactions (like the catalytic ozone depletion reactions alone). Prior to its discovery the scientific consensus was that the effect of ODS, such as CFCs, would be first seen near 40 km where the natural variability of ozone is relatively small. Since the ozone amount near 40 km is small, the total ozone column above the surface was expected to change very little. The globally averaged change in total column ozone was expected to be only about 1%/decade. Given the large natural variability of the total ozone, and the difficulty of maintaining long-term calibration of instruments to such high accuracy, such changes were not expected to be seen in the data for a decade or longer (Barthia & McPeters, 2018).
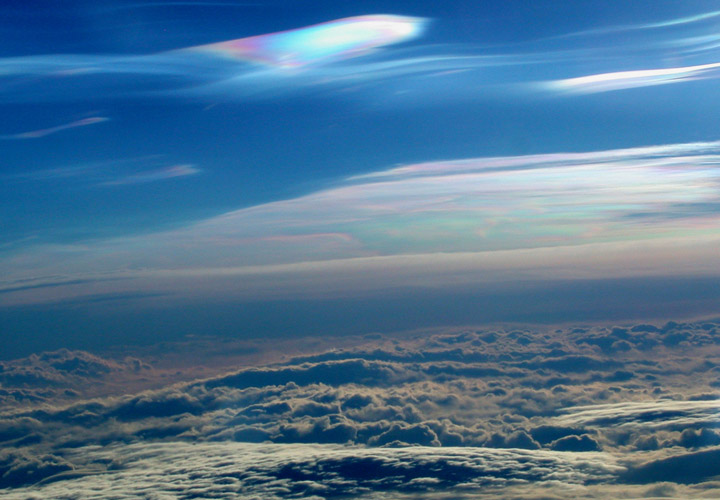
Between 1986 and 1987, several papers suggested possible mechanisms for the ozone hole, including chemical, dynamical (meteorological), and solar cycle influences. Among the key papers explaining the atmospheric chemistry of CFCs and ozone depletion was the Nature paper by Susan Solomon and several colleagues, emphasizing the need for polar stratospheric clouds to explain the reaction chemistry. Paul Crutzen and Frank Arnold proposed in their Nature paper that the polar stratospheric clouds could be made of nitric acid trihydrate, which would explain the clouds’ presence at an altitude and temperature that should not have been cold enough for the tiny amount of pure water vapor present in the stratosphere to condense. They also proposed that catalytic reactions of ClOx and BrOx might cause drastic ozone destructions and can account for the springtime ‘ozone hole’.
In the late 1980s, several research aircrafts flew into the springtime Antarctic stratosphere and finally provided the “smoking gun” linking CFC-derived chlorine to the ozone hole. The flight data showed a negative correlation between chlorine monoxide (ClO) and ozone: the higher the concentration of ClO, the lower the concentration of ozone. In 1988, Mario Molina and colleagues described the chemical reactions through which ClO catalyzes the extremely rapid destruction of ozone.
We explain here in a nutshell the mechanisms behind the formation of the ozone hole in the Antarctic. During the long dark (no sunlight!) Antarctic winter, stratospheric winds move in a circular pattern over the polar region, isolating the air above the Antarctic land mass and creating a polar vortex. Within the polar vortex, the stratospheric temperatures can become extremely low (around -80°C) and polar stratospheric clouds (PSCs, see Fig. 2) can be formed at altitudes between 15 and 25 km. Also CFCs, present globally in the atmosphere, gather here. Reactions on the surfaces of liquid water droplets or solid ice particles of the PSCs increase the relative abundances of the most reactive chlorine gases (converting the reservoir forms to the most reactive forms). For instance, the species Cl2 and HOCl are formed. These chlorine species are short lived and are quickly split up by sunlight (even in visible wavelengths) when the sun returns to the Antarctic in the early spring. The freed Cl and ClO species act as catalysts in chemical reaction cycles, destroying ozone in the stratosphere. So, the key ingredients to have ozone loss at the poles are (i) chlorine (ClO catalytic cycle), (ii) cold (polar vortex), (iii) seasons (dark and light), (iv) clouds (PSCs), and (v) UV radiation (springtime sunlight).
The Antarctic ozone hole
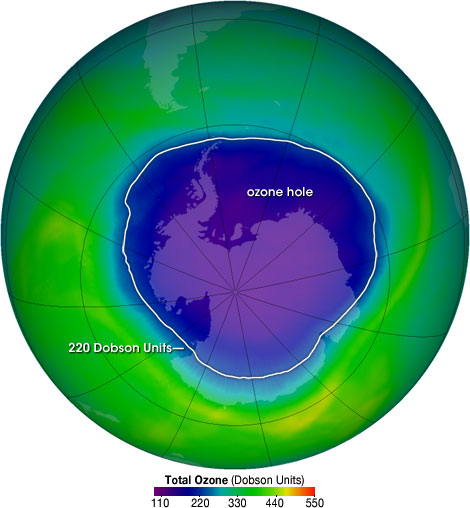
The ozone hole is not technically a “hole” where no ozone is present, but is actually a region of exceptionally depleted ozone in the stratosphere over the polar regions. Usually, the ozone hole area is marked by total ozone values lower than 220 Dobson Units (DU) (the white line in Fig. 3). This value is chosen because of two reasons. First, from the historical record we know that total column ozone values of less than 220 DU were not observed prior to 1979 (the beginning of satellite total ozone retrievals). Secondly, an aircraft field mission over Antarctica demonstrated that a total column ozone level of less than 220 DU is a result of catalytic ozone loss from chlorine and bromine compounds.
The time evolution of the ozone amounts above the Antarctic is shown in Fig. 4. In the 1970s, no ozone hole was observed. The ozone hole initially appeared in the early 1980s and increased in size until the early 1990s. Since 1987, when the Montreal Protocol on Substances that Deplete the Ozone Layer was signed, the ozone hole has stabilized to an area of about 20-25 million square kilometers (average value for the period 7 September - 13 October), with a maximum value reached of 27 million square kilometers in 2006. Notable exceptions are the years 2002 (12.0) and 2019 (9.3), due to a breaking up of the polar vortex and a very weak polar vortex with very high stratospheric temperatures, respectively. The thickness of the ozone layer in the ozone hole can be as low as 100 DU while it should be between 300 and 400 DU at that time (21 September–16 October). The minimum value reached was 92 DU in 1994. During the most recent years, values around 120 DU were measured. A notable exception is again 2019, with a high value of 167 DU. In 2020, the ozone hole was very persistent in time, being present until almost the end of December!
Because of the long lifetime of ODS in the atmosphere (up to 50-100 years!), the latest WMO/UN Environment Programme Scientific Assessment of Ozone Depletion, issued in 2018, concluded that the ozone layer is on the path of recovery and will potentially return of the ozone values over Antarctica to pre-1980 levels by 2060.
Fig. 4: Long-term changes in Antarctic total ozone are demonstrated with this series of total ozone maps derived from satellite observations. [Ref: Ross J. Salawitch (Lead Author) et al., "Twenty Questions and Answers About the Ozone Layer: 2022 Update", Scientific Assessment of Ozone Depletion: 2022, 75 pp., World Meteorological Organization, Geneva, Switzerland, 2023.]
An Arctic ozone hole?
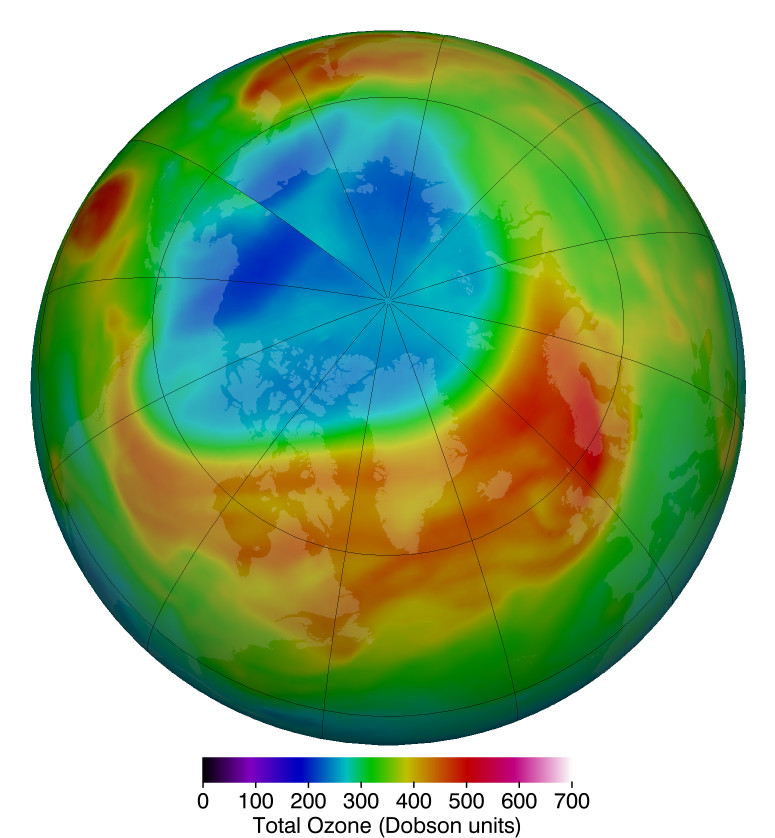
The specific chemical and dynamical conditions that favour the formation of the ozone hole above Antarctica, might be theoretically also fulfilled at the Arctic. However, the Arctic ozone depletion is not extensive and recurrent as the Antartic ozone hole because, in comparison to Antarctic conditions, average temperatures in the Arctic stratosphere are always significantly higher and the isolation of polar stratospheric air is less effective. These differences occur because Northern polar latitudes have more land and mountainous regions than Southern polar latitudes, which creates more meteorological disturbances that warm the Arctic stratosphere.
However, in 2020 the Arctic polar vortex has been exceptionally strong and long lived. Temperatures in the Arctic stratosphere were low enough for several months at the start of 2020 to allow the formation of PSCs, resulting in large ozone losses over the Arctic. The ozone values in Arctic reached a record low for the month of March, decreasing to less than 220 DU, which is normally considered ‘ozone hole levels,’ and at the peak down to 205 Dobson units. The typical lowest ozone values observed over the Arctic in March are at least 240 Dobson Units. The Arctic ozone hole closed in April with an increase in stratospheric temperatures which culminated in an influx of ozone-rich air from the lower atmosphere.
More information
Copernicus Monitoring of the ozone layer
Twenty questions and answers about the ozone layer: 2018 update